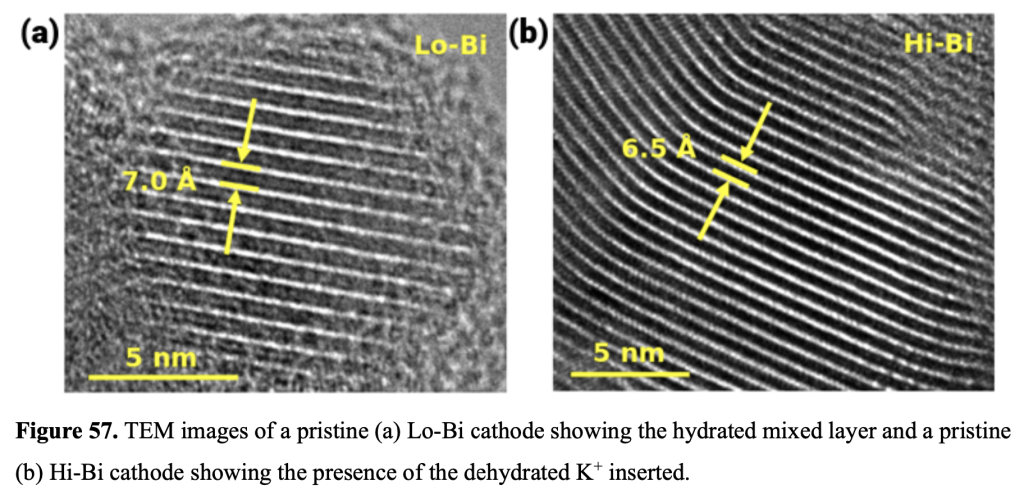
I had a reason to look at Matt Kim’s PhD dissertation, which was about synthesis of layered MnO2 birnessite compounds for Li-ion and Na-ion batteries. We published his work in this paper, but at the end of his dissertation I saw this image that didn’t make it into the paper. On the left you see a regular birnessite with a 7 angstrom interlayer, and on the right you see a dehydrated version, which is smaller because it is dehydrated and the interlayer H2O is gone.
I was looking at this and I was amazed because on the left I can see the H2O molecules between the layers. On the right you see black space only. Kind of a work of art. Matt, I’m sorry I left this out of the paper. Cutting the 60 figures down into a manuscript wasn’t the most orderly process, and I really should have left this one in. 🙂