Graduate
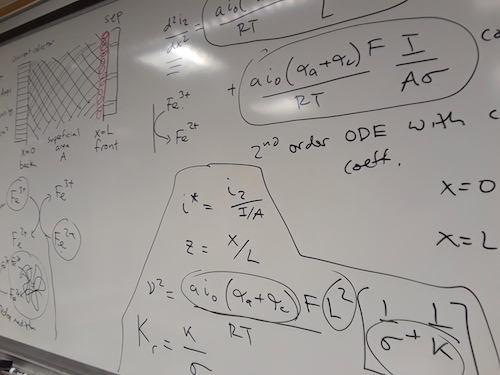
Electrochemical Engineering
CHME 5621, Northeastern University
Electrochemistry is fundamentally an interfacial science, dealing with reactions that happen between an electrode and electrolyte. At this interface, there is a nm-scale separation of charge (the double layer) and a micron-scale concentration gradient (the mass transport boundary layer). Cell performance may be limited by reaction kinetics across the double layer or transport phenomena across the boundary layer. This class is almost always about figuring out which one. Through the lens of chemical engineering fundamentals, we discuss batteries, fuel cells, electrodeposition, and the process of corrosion.
Undergraduate
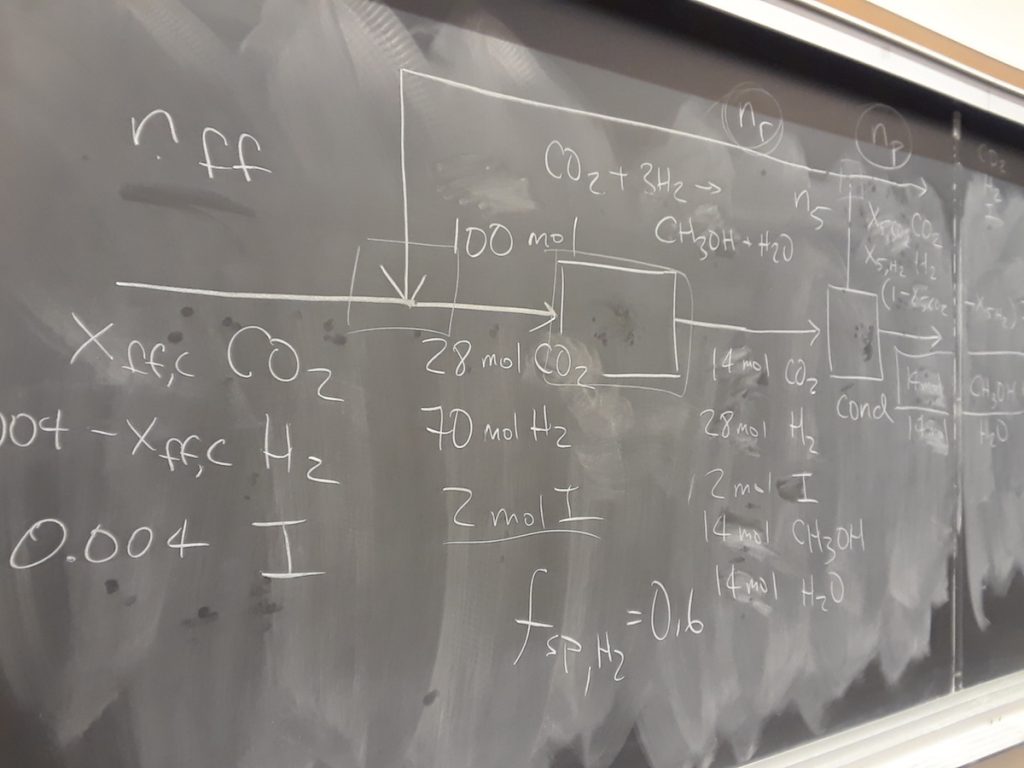
Conservation Principles
CHME 2308, Northeastern University
What does it mean to be a chemical engineer? This is the class where we begin to address that. It has to do with relating systems of algebraic equations to situations found in the real world. Once established, this coupling between the world and mathematics allows us to analyze processes, using two important concepts: conservation of mass and conservation of energy. What is a process? Lots of things qualify as “processes,” and that is another major topic we address.